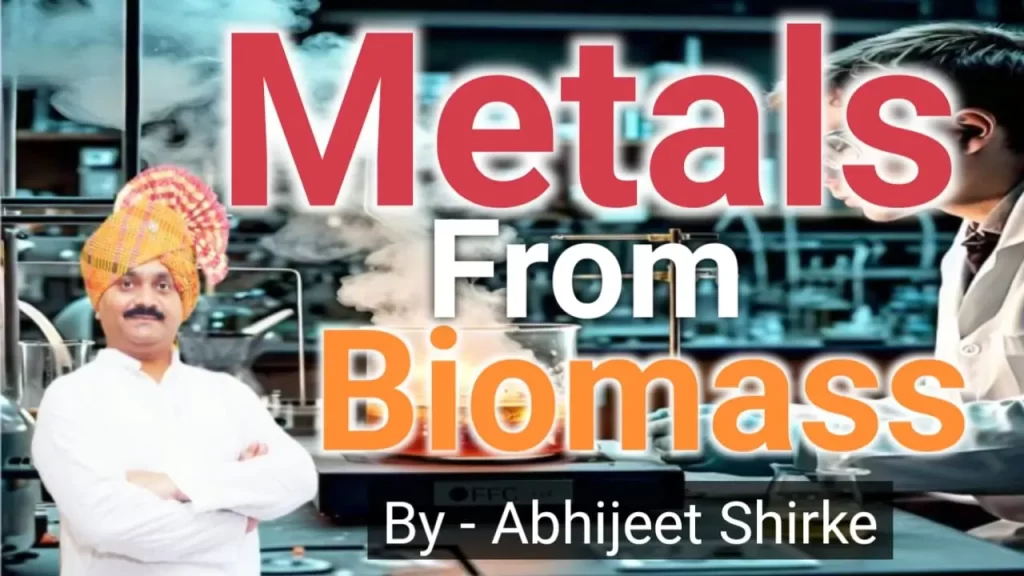
As the world shifts towards a more sustainable and environmentally conscious future, innovative solutions are being sought to reduce our reliance on traditional metal extraction methods. One such solution is the extraction of metals from biomass, a process that harnesses the power of organic matter to produce valuable metals. Biomass, comprising plant and animal waste, has long been recognized as a renewable energy source. However, its potential extends far beyond energy production. Recent advancements in biotechnology and materials science have unlocked the possibility of extracting metals from biomass, offering a promising alternative to conventional mining and smelting processes.
This groundbreaking approach not only reduces the environmental impact associated with traditional metal extraction but also provides a unique opportunity to recover valuable metals from waste streams. Metals such as copper, zinc, and nickel can be extracted from biomass through various biological and chemical processes, including bioleaching, biosorption, and pyrometallurgy. These methods leverage microorganisms, enzymes, and other biological agents to break down biomass and release the embedded metals.
The benefits of extracting metals from biomass are multifaceted. Firstly, it reduces the ecological footprint of metal production by minimizing the need for land excavation, water usage, and energy consumption. Secondly, it provides a closed-loop system where waste biomass is converted into valuable resources, promoting a circular economy. Lastly, this approach can help meet the increasing demand for metals in various industries, including renewable energy technologies, electronics, and construction.
As the world continues to grapple with the challenges of sustainable development, the extraction of metals from biomass presents a beacon of hope. This innovative field has the potential to transform the way we produce and consume metals, paving the way for a more environmentally conscious and resource-efficient future.
In this article, we will delve into the processes, applications, and future prospects of extracting metals from biomass, exploring the vast potential of this emerging field.
1. Biomass Can Absorb Metals from Soil and Water
Biomass, consisting of organic matter such as plants, algae, or agricultural waste, has a unique ability to absorb metals from the soil and water. This capability stems from the natural processes of phytoremediation, where plants uptake and sequester various contaminants, including heavy metals, through their root systems. The absorption of metals by plants is influenced by factors such as soil pH, organic matter content, and the presence of metal chelators.
Plants use their root systems to absorb nutrients and water from the soil, and during this process, they can also take up metals present in the environment. These metals can then be trans located to different parts of the plant, including the stems, leaves, and seeds. This ability is particularly significant in areas contaminated with metals due to industrial activities, mining, or agricultural practices. By absorbing these metals, plants help in cleaning up the environment while also accumulating valuable resources that can be extracted later.
The process of metal absorption by biomass is not uniform across all plant species. Some plants are better at absorbing specific metals than others. For example, Hyperaccumulator plants are particularly efficient at absorbing and concentrating metals like nickel, cadmium, and zinc. These plants have evolved mechanisms to tolerate high levels of metals that would be toxic to most other species.
Understanding the mechanisms behind metal absorption by biomass is crucial for optimizing the process for industrial applications. Researchers are studying the genetic and biochemical pathways involved in metal uptake and accumulation to enhance the efficiency of these natural processes. By identifying key genes and proteins involved in metal transport and sequestration, scientists can develop genetically modified plants with improved capabilities for phytoremediation and metal extraction.
The ability of biomass to absorb metals from soil and water is a natural process that can be harnessed for environmental cleanup and metal extraction. This process not only helps in reducing metal pollution in the environment but also provides a sustainable source of valuable metals that can be used in various industries.
2. Certain Plants, Like Hyperaccumulators, Can Concentrate High Levels of Metals
Hyperaccumulator plants are a unique group of plants capable of absorbing and concentrating exceptionally high levels of metals in their tissues, often to concentrations hundreds or even thousands of times higher than those found in non-accumulating species. This extraordinary ability makes Hyperaccumulators invaluable for the process of Phytomining or Agromining, where they are used to extract metals from soil or water in an environmentally friendly manner.
These plants have evolved specialized mechanisms that allow them to tolerate, sequester, and detoxify metals that are typically harmful to other organisms. The primary mechanisms include:
- Metal Uptake and Transport: Hyperaccumulators possess root systems that efficiently take up metals from the soil. Once absorbed, these metals are transported to various parts of the plant through specialized transport proteins located in the cell membranes.
- Metal Chelation and Sequestration: Within the plant tissues, metals are often bound to organic molecules called chelators, which render them less toxic. These chelated metals are then sequestered in cellular compartments such as vacuoles, where they are stored safely away from vital cellular processes.
- Detoxification Pathways: Hyperaccumulators have robust detoxification pathways that include the production of antioxidants and other protective molecules that mitigate the oxidative stress caused by high metal concentrations.
Some well-known Hyperaccumulator plants include Alyssum murale and Thlaspicaerulescens, which are particularly adept at accumulating nickel and zinc, respectively. These plants are often found in naturally metal-rich soils, known as serpentine soils, where they thrive despite the high metal content that would be toxic to most other plant species.
The ability of Hyperaccumulator plants to concentrate metals has several practical applications. In addition to Phytomining, these plants are used in phytoremediation projects to clean up contaminated sites. By planting Hyperaccumulators in polluted areas, metals can be extracted from the soil and stored in the plant biomass, which can then be harvested and processed to recover the metals. This process not only cleans the environment but also provides a source of valuable metals.
Research into Hyperaccumulator plants is ongoing, with scientists exploring ways to enhance their metal uptake capabilities through genetic engineering and selective breeding. By identifying and manipulating the genes responsible for metal uptake, transport, and sequestration, researchers aim to develop super-accumulators with even greater efficiency and broader applicability.
Hyperaccumulator plants are a remarkable natural resource for the extraction and remediation of metals from the environment. Their unique abilities offer sustainable and eco-friendly alternatives to traditional mining and pollution cleanup methods, making them an essential tool in the quest for sustainable development and environmental conservation.
3. Metals from Biomass Can Be Extracted Through Various Processes
Extracting metals from biomass involves a series of processes that separate the metals from the organic matter and refine them into usable forms. These processes, collectively known as Phytomining or Agromining, leverage the natural metal-accumulating abilities of certain plants to provide an eco-friendly alternative to conventional mining. The key steps in the extraction process include biomass harvesting, drying, ashing, and metal recovery.
a) Biomass Harvesting: The first step in extracting metals from biomass is harvesting the plants that have accumulated metals in their tissues. This typically involves cutting the above-ground parts of the plants, such as leaves and stems, which contain the highest concentrations of metals. The harvested biomass is then transported to a processing facility.
b) Drying and Ashing: Once harvested, the biomass is dried to remove moisture, making it easier to handle and process. Drying can be done using natural sunlight or industrial drying equipment. After drying, the biomass is subjected to ashing, where it is burned at high temperatures to convert the organic matter into ash. This process concentrates the metals in the ash, significantly reducing the volume of material that needs to be processed further.
c) Metal Recovery: The ash, now rich in metals, undergoes various chemical and physical treatments to extract and purify the metals. Common methods for metal recovery include:
i) Leaching: Using solvents such as acids or bases to dissolve the metals from the ash, followed by precipitation or electrochemical processes to recover the metals from the solution.
ii) Bioleaching: Utilizing microorganisms to bio-convert metal compounds into soluble forms, which are then recovered through similar precipitation or electrochemical methods.
iii) Hydrometallurgy: Employing aqueous chemistry techniques to extract metals from the ash, including solvent extraction, ion exchange, and electrowinning.
d) Purification and Refining: The recovered metals often require further purification to achieve the desired level of purity. This may involve additional chemical treatments, smelting, or electrorefining processes to remove impurities and produce high-purity metal products suitable for industrial applications.
Each of these processes can be optimized depending on the type of metal being extracted and the specific characteristics of the biomass. For example, the extraction of nickel from Hyperaccumulator plants like Alyssum murale involves specific leaching and precipitation techniques tailored to nickel compounds.
The development and refinement of these extraction processes are critical to making Phytomining economically viable and efficient. Researchers are continually exploring new methods and technologies to enhance metal recovery rates, reduce costs, and minimize environmental impacts. Innovations such as the use of advanced biotechnologies and green chemistry principles are paving the way for more sustainable and scalable Phytomining practices.
In summary, extracting metals from biomass involves a series of well-coordinated processes designed to efficiently recover valuable metals from plant tissues. These methods offer a promising and environmentally friendly alternative to traditional mining, contributing to the sustainable production of metals while promoting environmental remediation and resource conservation.
4. Phytomining Can Be a More Environmentally Friendly Alternative to Traditional Mining
Phytomining, the process of extracting metals from biomass, offers a sustainable and environmentally friendly alternative to traditional mining practices. Conventional mining often involves extensive excavation, the use of heavy machinery, and significant disruption of ecosystems, leading to various environmental issues such as deforestation, habitat destruction, soil erosion, and water pollution. In contrast, Phytomining leverages the natural abilities of certain plants to absorb and concentrate metals from the soil, offering a less invasive and more sustainable approach to metal extraction.
One of the primary environmental benefits of Phytomining is its potential to reduce the ecological footprint of mining activities. By using plants to extract metals, Phytomining eliminates the need for large-scale excavation and the associated destruction of landscapes. This approach helps preserve natural habitats and biodiversity, protecting ecosystems that would otherwise be severely impacted by traditional mining operations.
Phytomining also addresses the issue of soil and water pollution. Traditional mining often generates large amounts of waste, including tailings and sludge, which can leach harmful chemicals and heavy metals into the environment, contaminating soil and water sources. Phytomining, on the other hand, utilizes plants that can remediate contaminated sites by absorbing and sequestering metals from the soil. This dual function of metal extraction and environmental cleanup makes Phytomining a valuable tool for restoring polluted areas and improving soil and water quality.
Another significant advantage of Phytomining is its potential to reduce greenhouse gas emissions. Traditional mining processes, particularly those involving the extraction and processing of ores, are energy-intensive and contribute to significant carbon dioxide emissions. Phytomining, being less reliant on heavy machinery and extensive energy inputs, offers a lower-carbon alternative. Additionally, the plants used in Phytomining can sequester carbon dioxide through photosynthesis, further mitigating the carbon footprint of metal extraction.
The use of Phytomining can also promote sustainable land management practices. By integrating metal-accumulating plants into agricultural systems, farmers can use Phytomining as a supplementary income source while maintaining or improving soil health. This practice supports sustainable 4. Phytomining Can Be a More Environmentally Friendly Alternative to Traditional Mining land use and provides economic benefits without compromising agricultural productivity. By choosing crops that are both valuable for Phytomining and suitable for intercropping or rotation, farmers can enhance soil fertility and reduce the need for chemical fertilizers.
Furthermore, Phytomining can be particularly advantageous in regions where traditional mining is impractical or economically unviable due to low ore concentrations or challenging terrain. In such areas, planting Hyperaccumulator species can provide a cost-effective means of metal recovery, turning otherwise unusable land into productive sites for metal extraction. This can be especially beneficial for developing countries seeking to harness their natural resources in an environmentally responsible manner.
Despite its numerous benefits, Phytomining also faces challenges that need to be addressed to achieve widespread adoption. These include optimizing plant species selection, improving metal uptake efficiency, and developing cost-effective harvesting and processing methods. Ongoing research is essential to overcoming these obstacles and making Phytomining a viable and scalable alternative to traditional mining.
Phytomining represents a promising, environmentally friendly approach to metal extraction that minimizes ecological disruption, promotes soil and water remediation, reduces greenhouse gas emissions, and supports sustainable land management practices. By harnessing the natural capabilities of Hyperaccumulator plants, Phytomining offers a sustainable solution to the environmental challenges associated with conventional mining, paving the way for a greener and more sustainable future.
5. Agromining Can Provide an Additional Income Stream for Farmers
Agromining, the practice of using Hyperaccumulator plants to extract metals from the soil, offers a novel and sustainable way for farmers to generate additional income. This approach integrates metal recovery into agricultural systems, providing farmers with a supplementary revenue stream without compromising traditional crop production. By adopting Agromining, farmers can diversify their income sources, enhance the economic viability of their operations, and contribute to environmental sustainability.
One of the primary advantages of Agromining is the potential for farmers to profit from metal-rich soils that would otherwise be unsuitable for conventional Agriculture. In regions with naturally occurring high concentrations of metals or areas contaminated by industrial activities, traditional crops may not thrive, and soil remediation can be costly. However, by cultivating Hyperaccumulator plants, farmers can turn these challenging environments into productive lands. The harvested biomass, enriched with valuable metals, can be processed and sold to metal recovery industries, providing a new income source.
Additionally, Agromining can be integrated into existing agricultural practices, allowing farmers to optimize land use and increase overall productivity. For example, Hyperaccumulator plants can be grown in rotation with traditional crops, enhancing soil health and reducing the need for chemical inputs. This practice not only supports sustainable Agriculture but also provides a steady flow of metal-enriched biomass that can be harvested and processed regularly.
Moreover, Agromining can contribute to local economies by creating new job opportunities in rural areas. The cultivation, harvesting, and processing of Hyperaccumulator plants require labor, which can stimulate employment and support community development. As the Agromining industry grows, it can also attract investment and foster the establishment of related businesses, such as processing facilities and logistics services, further boosting economic activity in agricultural regions.
The economic benefits of Agromining extend beyond individual farmers to the broader agricultural sector. By providing a new revenue stream, Agromining can help stabilize farm incomes, reducing the financial risks associated with fluctuating commodity prices and adverse weather conditions. This stability is particularly important for small-scale farmers who are often more vulnerable to economic shocks. By diversifying their income sources through Agromining, farmers can achieve greater financial resilience and sustainability.
However, the successful implementation of Agromining requires careful planning and support. Farmers need access to information and resources to select appropriate Hyperaccumulator species, manage soil health, and optimize harvesting and processing techniques. Collaboration between agricultural researchers, extension services, and farmers is crucial to developing best practices and ensuring the economic viability of Agromining.
In conclusion, Agromining presents a unique opportunity for farmers to generate additional income by leveraging the natural abilities of Hyperaccumulator plants. This innovative practice not only provides financial benefits but also supports sustainable land use, environmental remediation, and community development. By integrating Agromining into agricultural systems, farmers can enhance their economic resilience and contribute to a more sustainable and diversified agricultural sector.
6. Biomass Can Be Used to Clean Polluted Soil and Water
Biomass, particularly from Hyperaccumulator plants, can play a crucial role in cleaning polluted soil and water through a process known as phytoremediation. This environmentally friendly technique uses plants to remove, transfer, stabilize, or destroy contaminants in the environment, offering a natural and sustainable solution for pollution management. Phytoremediation can be applied to a variety of contaminants, including heavy metals, organic pollutants, and radionuclides, making it a versatile tool for environmental cleanup.
One of the key advantages of using biomass for phytoremediation is its ability to extract heavy metals from contaminated soils. Hyperaccumulator plants, which can tolerate and concentrate high levels of metals in their tissues, are particularly effective for this purpose. When planted in polluted areas, these plants absorb metals through their root systems and store them in their leaves, stems, and roots. Over time, repeated planting and harvesting of Hyperaccumulators can significantly reduce metal concentrations in the soil, leading to gradual detoxification of the contaminated site.
Phytoremediation is also applicable to water bodies contaminated with heavy metals and other pollutants. Aquatic plants, such as water hyacinth and duckweed, are capable of absorbing contaminants from water through their roots and shoots. These plants can be used to treat polluted ponds, lakes, and wastewater, removing harmful substances and improving water quality. The harvested biomass can then be processed to recover the absorbed metals, providing a source of valuable materials while cleaning the water.
In addition to heavy metals, biomass can also be used to remediate organic pollutants. Certain plants have the ability to break down and degrade organic contaminants, such as pesticides, hydrocarbons, and solvents, through metabolic processes. This form of phytoremediation, known as phytodegradation, involves the uptake of organic pollutants by plants, followed by their transformation into less harmful compounds. This process helps in detoxifying the environment and preventing the accumulation of hazardous substances in the ecosystem.
Phytoremediation offers several environmental and economic benefits compared to traditional remediation methods. It is a cost-effective and sustainable approach that utilizes natural processes to clean up contaminated sites. Unlike physical or chemical remediation techniques, which can be expensive and disruptive, phytoremediation is relatively low-cost and minimally invasive. It also enhances soil structure and fertility, promoting the restoration of ecosystems and supporting biodiversity.
However, the effectiveness of phytoremediation depends on various factors, including the type and concentration of contaminants, soil and water conditions, and the selection of appropriate plant species. Research is ongoing to identify and develop plants with enhanced phytoremediation capabilities, optimize planting and harvesting techniques, and improve the overall efficiency of the process.
In conclusion, biomass, particularly from Hyperaccumulator and aquatic plants, offers a sustainable and eco-friendly solution for cleaning polluted soil and water through phytoremediation. This natural process not only helps in removing contaminants from the environment but also supports the recovery of valuable metals and promotes ecological restoration. By harnessing the power of plants, phytoremediation provides a promising approach to managing environmental pollution and fostering a cleaner and healthier planet.
7. Metals Extracted from Biomass Can Be Used in Various Industries
The metals extracted from biomass through Phytomining and Agromining processes hold significant potential for various industrial applications. These metals, recovered in an environmentally friendly manner, can be utilized across a wide range of sectors, contributing to the sustainable development of industries while reducing the environmental impact of traditional metal extraction methods.
One of the primary industries that can benefit from metals extracted from biomass is the electronics industry. Metals such as nickel, copper, and gold are essential components in the manufacturing of electronic devices, including smartphones, computers, and circuit boards. Phytomining provides a sustainable source of these metals, reducing the reliance on traditional mining and the associated environmental degradation. The high purity of metals recovered from Hyperaccumulator plants makes them suitable for precision applications in the electronics sector.
The automotive industry also stands to gain from metals extracted from biomass. Metals like nickel and copper are crucial for the production of batteries, electric motors, and wiring systems in electric and hybrid vehicles. As the demand for electric vehicles continues to grow, sourcing metals sustainably through Phytomining can help meet this demand while minimizing the environmental footprint. Additionally, using metals from biomass can support the development of lighter and more efficient vehicles, contributing to improved fuel efficiency and reduced emissions.
In the energy sector, metals recovered from biomass can be used in the production of renewable energy technologies. For instance, copper and nickel are essential components in wind turbines, solar panels, and energy storage systems. By providing a sustainable source of these metals, Phytomining can support the expansion of renewable energy infrastructure and promote the transition to cleaner energy sources. The integration of sustainably sourced metals into renewable energy technologies aligns with the goals of reducing greenhouse gas emissions and combating climate change.
The construction industry can also benefit from metals extracted from biomass. Metals such as zinc and nickel are used in galvanizing steel, a process that protects steel structures from corrosion and extends their lifespan. Sustainable sourcing of these metals through Phytomining can reduce the environmental impact of construction activities and support the development of more durable and sustainable infrastructure. Moreover, the use of metals from biomass can contribute to the circular economy by promoting the recycling and reuse of materials in the construction sector.
The medical industry is another sector where metals from biomass can be applied. Metals like gold and copper have antimicrobial properties, making them valuable for medical devices, implants, and coatings. The sustainable extraction of these metals through Phytomining ensures a reliable supply for medical applications while minimizing the environmental impact. Additionally, the use of biocompatible metals sourced from plants can enhance the safety and effectiveness of medical devices.
In summary, the utilization of metals extracted from biomass can significantly benefit a variety of industries, promoting sustainability and environmental responsibility.
8. Research is Ongoing to Improve Efficiency and Scalability
The field of Phytomining and Agromining is still in its nascent stages, and extensive research is being conducted to enhance the efficiency and scalability of these processes. Scientists and engineers are focusing on various aspects, from the selection of optimal Hyperaccumulator species to the development of advanced extraction and processing technologies.
One area of research is the identification and genetic improvement of Hyperaccumulator plants. Scientists are exploring the genetic mechanisms that enable certain plants to absorb and tolerate high levels of metals. By understanding these mechanisms, researchers can potentially develop genetically modified plants with enhanced metal uptake capabilities. This could lead to higher yields of metal-rich biomass and improve the economic viability of Phytomining operations.
Another critical area of research is optimizing soil and environmental conditions to maximize metal uptake by plants. Factors such as soil pH, nutrient levels, and microbial interactions can significantly influence the efficiency of metal accumulation in plants. Researchers are studying these factors to develop best practices for cultivating Hyperaccumulator plants in various environments. This includes exploring the use of soil amendments and microbial inoculants to enhance metal uptake and plant growth.
Advancements in processing technologies are also crucial for the scalability of Phytomining. Efficient methods for harvesting, drying, and processing metal-rich biomass are essential to recover metals cost-effectively. Researchers are developing innovative extraction techniques, such as bioleaching and Phytomining-specific smelting processes, to improve the recovery rates and purity of extracted metals. Additionally, integrating Phytomining with other agricultural and industrial processes can create synergies that enhance overall efficiency.
Economic viability is a significant challenge in Phytomining, and ongoing research aims to address this by improving the cost-effectiveness of the entire value chain. This includes reducing the costs of cultivation, harvesting, and processing, as well as identifying high-value applications for extracted metals. Collaborations between researchers, industry stakeholders, and policymakers are essential to create supportive frameworks that incentivize investment in Phytomining technologies.
Furthermore, researchers are exploring the potential of Phytomining in different geographical and environmental contexts. Pilot projects and field trials are being conducted in various regions to assess the feasibility and effectiveness of Phytomining in different soil types and climatic conditions. These studies provide valuable insights into the practical challenges and opportunities associated with large-scale implementation of Phytomining.
In conclusion, ongoing research in Phytomining and Agromining is focused on enhancing the efficiency, scalability, and economic viability of these sustainable metal extraction methods. By advancing our understanding of plant biology, soil science, and processing technologies, researchers are paving the way for the widespread adoption of Phytomining as a viable alternative to traditional mining. This research not only contributes to sustainable metal production but also supports environmental remediation and agricultural development.
9. Challenges Include Optimizing Extraction Processes and Ensuring Economic Viability
Despite the promising potential of Phytomining and Agromining, several challenges must be addressed to optimize extraction processes and ensure economic viability. These challenges span across various stages of the value chain, from plant cultivation to metal recovery and market integration.
One of the primary challenges is the optimization of plant species and cultivation practices. Not all Hyperaccumulator plants are suitable for all environments, and selecting the right species for specific soil and climatic conditions is critical. Researchers must identify and develop plants that can thrive in diverse conditions while efficiently accumulating target metals. This involves extensive field trials, genetic studies, and breeding programs to produce plants with enhanced metal uptake capabilities and resilience to environmental stressors.
Soil management is another critical aspect that influences the success of Phytomining. Soil characteristics, such as pH, nutrient levels, and the presence of competing contaminants, can impact metal availability and plant uptake. Developing effective soil management practices, including the use of amendments and microbial inoculants, is essential to optimize metal bioavailability and enhance plant growth. Additionally, managing the potential ecological impacts of introducing Hyperaccumulator species into new environments requires careful consideration and monitoring.
The extraction and processing of metals from plant biomass pose technical and economic challenges. Efficient methods for harvesting, drying, and processing metal-rich biomass are necessary to recover metals in a cost-effective manner. Traditional extraction methods, such as smelting and leaching, may not be suitable for Phytomining due to the unique composition of plant biomass. Developing specialized extraction technologies that can handle the organic matrix of plant material while maximizing metal recovery is a key research focus. Additionally, ensuring that these technologies are economically viable and scalable is crucial for the widespread adoption of Phytomining.
Economic viability is a significant hurdle for Phytomining. The costs associated with plant cultivation, harvesting, and processing must be offset by the value of the recovered metals. This requires identifying high-value applications for extracted metals and creating market demand. Collaborations between researchers, industry stakeholders, and policymakers are essential to develop supportive frameworks that incentivize investment in Phytomining technologies. This includes exploring potential subsidies, tax incentives, and other financial mechanisms that can make Phytomining more attractive to investors and farmers.
Market integration of metals extracted from biomass is another challenge. Establishing reliable supply chains and market channels for these metals is essential to ensure their economic viability. This involves developing standards and certifications for metals sourced through Phytomining, creating awareness and demand among end-users, and establishing partnerships with industries that can utilize these metals. Building a robust market infrastructure that can support the commercialization of Phytomining is critical for its long-term success.
In summary, optimizing extraction processes and ensuring economic viability are key challenges that need to be addressed for the successful implementation of Phytomining and Agromining. By focusing on plant selection, soil management, extraction technologies, and market integration, researchers and stakeholders can overcome these challenges and unlock the full potential of sustainable metal extraction from biomass. This will not only provide a viable alternative to traditional mining but also contribute to environmental sustainability and economic development.
10. Metals from Biomass Can Contribute to a Circular Economy
The extraction of metals from biomass through Phytomining and Agromining aligns with the principles of a circular economy, which emphasizes resource efficiency, waste reduction, and the sustainable use of natural resources. By integrating metal recovery from biomass into industrial processes, we can create closed-loop systems that minimize waste, reduce environmental impact, and promote the sustainable use of metals.
One of the fundamental principles of a circular economy is the efficient use of resources. Phytomining and Agromining contribute to this by utilizing plants to extract metals from soil and water, turning organic waste into valuable materials. This process reduces the need for traditional mining, which often involves significant environmental degradation and resource consumption. By recovering metals from biomass, we can make more efficient use of existing resources and reduce the overall demand for virgin metals.
Another key aspect of a circular economy is waste reduction. Phytomining and Agromining can help address this by transforming waste materials, such as agricultural residues and contaminated soils, into sources of valuable metals. This not only reduces the amount of waste that needs to be managed but also provides an additional revenue stream for farmers and landowners. By converting waste into a resource, Phytomining supports the circular economy goal of minimizing waste and maximizing value.
The metals recovered from biomass can be reintegrated into various industrial processes, creating closed-loop systems that promote sustainability. For example, metals such as nickel, copper, and zinc, which are commonly extracted through Phytomining, can be used in manufacturing, electronics, and construction. By sourcing these metals from biomass, industries can reduce their reliance on traditional mining and decrease their environmental footprint. This closed-loop approach ensures that metals are continuously recycled and reused, extending their lifecycle and reducing the need for new extraction.
Phytomining also supports the principles of a circular economy by promoting environmental remediation. Hyperaccumulator plants can be used to clean up contaminated soils and water bodies, removing pollutants and restoring ecosystem health. This process not only recovers valuable metals but also rehabilitates degraded environments, making them suitable for agricultural or other productive uses. By integrating environmental cleanup with metal recovery, Phytomining enhances the overall sustainability of land management practices.
The circular economy framework also emphasizes the importance of innovation and collaboration. Ongoing research and development in Phytomining are crucial to improving the efficiency and scalability of this technology. Collaboration between researchers, industry stakeholders, policymakers, and farmers is essential to create supportive frameworks that incentivize the adoption of Phytomining. By fostering innovation and cooperation, we can accelerate the transition to a circular economy and ensure the sustainable use of metals.
In conclusion, the extraction of metals from biomass through Phytomining and Agromining contributes to a circular economy by promoting resource efficiency, waste reduction, and the sustainable use of natural resources. By integrating metal recovery from biomass into industrial processes, we can create closed-loop systems that minimize environmental impact and support economic development. This innovative approach not only provides a sustainable solution for metal production but also enhances environmental remediation and agricultural sustainability, aligning with the goals of a circular economy.
Examples of Metals Extracted from Biomass
- Nickel from Hyperaccumulator Plants: Nickel is one of the most well-known metals that can be extracted from biomass, particularly from Hyperaccumulator plants such as Alyssum and Streptanthus. These plants have the remarkable ability to accumulate high levels of nickel in their tissues, making them ideal candidates for Phytomining. Nickel is a critical metal used in stainless steel production, batteries, and various industrial applications. By harvesting and processing nickel-rich biomass, we can produce nickel sustainably, reducing the environmental impact of traditional nickel mining.
- Gold from Algae: Gold can be extracted from biomass, particularly from certain species of algae. Some algae have the ability to bioaccumulate gold from water, making them valuable for biorecovery processes. This method of extracting gold is not only environmentally friendly but also cost-effective compared to traditional gold mining, which often involves harmful chemicals and significant environmental disruption. Gold recovered from algae can be used in electronics, jewelry, and medical applications.
- Copper from Agricultural Waste: Copper is an essential metal used in electrical wiring, plumbing, and various industrial applications. Agricultural waste, such as plant residues and manure, can be utilized to extract copper. Certain plants can accumulate copper from the soil, and their biomass can be processed to recover this metal. This approach not only provides a sustainable source of copper but also helps in managing agricultural waste. The extraction of copper from agricultural waste aligns with the principles of a circular economy by turning waste into a valuable resource.
- Zinc from Phytomining: Zinc is another metal that can be extracted through Phytomining. Hyperaccumulator plants like Thlaspicaerulescens are known for their ability to accumulate high levels of zinc. Zinc is widely used in galvanizing steel, making batteries, and producing alloys. By using Hyperaccumulator plants to extract zinc, we can reduce the environmental impact of traditional zinc mining and create a more sustainable supply chain for this essential metal.
Sustainable Solution for Metal Production and Environmental Remediation
The extraction of metals from biomass through Phytomining and Agromining offers a sustainable solution for metal production while simultaneously promoting environmental remediation and agricultural development. This innovative approach addresses several critical issues, including the environmental impact of traditional mining, the need for sustainable agricultural practices, and the importance of waste management.
- Environmental Impact: Traditional mining methods often involve significant environmental degradation, including deforestation, soil erosion, water pollution, and habitat destruction. Phytomining, on the other hand, is a much more environmentally friendly approach. By using plants to extract metals, we can minimize the need for large-scale mining operations, reducing the associated environmental footprint. Additionally, Phytomining can be used to remediate contaminated soils and water bodies, restoring ecosystems and promoting biodiversity.
- Sustainable Agriculture: Agromining can provide farmers with an additional income stream, making Agriculture more economically viable. By cultivating Hyperaccumulator plants, farmers can generate revenue from the sale of metal-rich biomass while also improving soil health and fertility. This dual benefit supports sustainable agricultural practices and helps farmers diversify their income sources. Moreover, integrating Phytomining with traditional crop production can enhance land use efficiency and promote sustainable land management.
- Waste Management: The utilization of agricultural waste and other organic materials for metal extraction aligns with the principles of waste management and circular economy. By converting waste into a valuable resource, Phytomining helps reduce the amount of waste that needs to be managed and minimizes the environmental impact of waste disposal. This approach also supports the recycling and reuse of materials, promoting a more sustainable and efficient use of resources.
- Research and Development: Ongoing research and development are critical to improving the efficiency, scalability, and economic viability of Phytomining and Agromining. Innovations in plant breeding, soil management, and extraction technologies are essential to optimize these processes and make them more commercially attractive. Collaboration between researchers, industry stakeholders, and policymakers is necessary to create supportive frameworks and incentives for the adoption of Phytomining technologies.
- Circular Economy: The extraction of metals from biomass supports the transition to a circular economy by promoting resource efficiency, waste reduction, and the sustainable use of natural resources. By creating closed-loop systems that integrate metal recovery from biomass into industrial processes, we can minimize environmental impact and support economic development. This approach not only provides a sustainable solution for metal production but also enhances environmental remediation and agricultural sustainability.
The extraction of metals from biomass through Phytomining and Agromining offers a promising and sustainable solution for metal production. By leveraging the natural ability of Hyperaccumulator plants to absorb and concentrate metals, we can reduce the environmental impact of traditional mining, support sustainable Agriculture, and promote waste management. Ongoing research and development are essential to optimize these processes and ensure their economic viability. The integration of Phytomining into a circular economy framework can create closed-loop systems that minimize waste, enhance resource efficiency, and support environmental and economic sustainability.
This innovative approach holds great potential for addressing some of the most pressing challenges of our time, providing a sustainable pathway for metal production, environmental remediation, and agricultural development. As the world shifts towards a more sustainable and environmentally conscious future, innovative solutions are being sought to reduce our reliance on traditional metal extraction methods. One such solution is the extraction of metals from biomass, a process that harnesses the power of organic matter to produce valuable metals.
Biomass, comprising plant and animal waste, has long been recognized as a renewable energy source. However, its potential extends far beyond energy production. Recent advancements in biotechnology and materials science have unlocked the possibility of extracting metals from biomass, offering a promising alternative to conventional mining and smelting processes.
This groundbreaking approach not only reduces the environmental impact associated with traditional metal extraction but also provides a unique opportunity to recover valuable metals from waste streams. Metals such as copper, zinc, and nickel can be extracted from biomass through various biological and chemical processes, including bioleaching, biosorption, and pyrometallurgy. These methods leverage microorganisms, enzymes, and other biological agents to break down biomass and release the embedded metals.
The benefits of extracting metals from biomass are multifaceted. Firstly, it reduces the ecological footprint of metal production by minimizing the need for land excavation, water usage, and energy consumption. Secondly, it provides a closed-loop system where waste biomass is converted into valuable resources, promoting a circular economy. Lastly, this approach can help meet the increasing demand for metals in various industries, including renewable energy technologies, electronics, and construction.