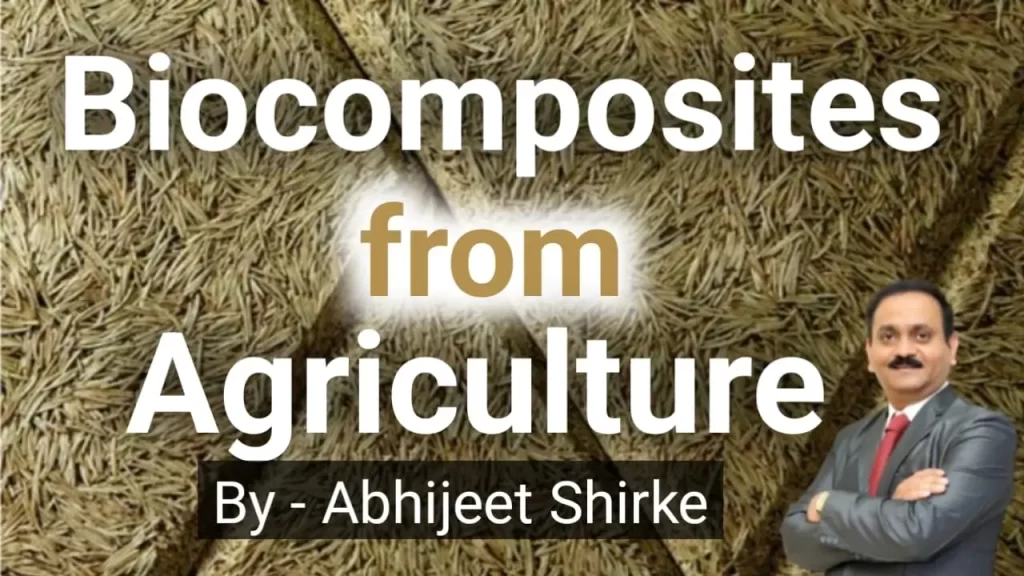
In recent years, the global push towards sustainability has led to significant advancements in various sectors, including materials science. Among these advancements, biocomposites have emerged as a promising innovation, combining the strengths of natural and synthetic materials. Biocomposites are composite materials where at least one of the constituents is derived from natural sources, typically agricultural by-products or residues. These materials not only offer environmental benefits but also hold potential for economic and social advancement, particularly in rural and agricultural communities.
Biocomposites from agriculture harness the abundant, renewable resources available in the agricultural sector, transforming them into valuable products. This process supports the circular economy by reducing waste and providing a sustainable alternative to traditional composites made from non-renewable resources. As the world grapples with the challenges of climate change, resource depletion, and environmental degradation, biocomposites present a new hope for a greener, more sustainable future.
Biocomposites are materials made by combining natural fibers with a matrix, which can be either natural or synthetic. The natural fibers used in biocomposites are typically derived from plants such as flax, hemp, jute, kenaf, and sisal. These fibers are abundant, renewable, and biodegradable, making them an excellent alternative to synthetic fibers like glass or carbon.
The agricultural sector produces vast amounts of biomass annually, much of which is considered waste or by-products. This biomass includes crop residues such as straw, husks, and stalks, as well as other plant materials. By utilizing these agricultural by-products in the production of biocomposites, we can reduce waste, lower the environmental impact of farming, and create new value streams for farmers.
Biocomposites have a wide range of applications across various industries, including automotive, construction, packaging, and consumer goods. Their use can lead to significant reductions in the carbon footprint of products, as well as improvements in sustainability and resource efficiency. Moreover, biocomposites often exhibit desirable properties such as high strength to weight ratios, thermal insulation, and biodegradability, making them competitive with traditional composite materials.
The Technology Behind Biocomposites
The production of biocomposites involves several key technological processes, including the selection and preparation of natural fibers, the choice of matrix materials, and the methods used to combine these components into a cohesive material.
Selection and Preparation of Natural Fibers
The first step in creating biocomposites is selecting suitable natural fibers. This selection is based on factors such as the availability of the fibers, their mechanical properties, and their compatibility with the chosen matrix material. Once selected, the fibers undergo a series of preparation steps to enhance their performance and ensure uniformity.
These preparation steps often include:
- Extraction and Cleaning: Natural fibers are extracted from plant materials through mechanical or chemical processes. The extracted fibers are then cleaned to remove impurities and improve their quality.
- Treatment: To enhance the compatibility between natural fibers and the matrix, the fibers may be treated with chemicals or enzymes. This treatment can improve adhesion, reduce moisture absorption, and enhance the mechanical properties of the fibers.
- Sizing and Processing: The fibers are then sized and processed into a form suitable for composite manufacturing, such as mats, yarns, or short fibers.
Choice of Matrix Materials
The matrix in a biocomposite binds the natural fibers together and transfers loads between them. The choice of matrix material is crucial for determining the final properties of the biocomposite. There are two main types of matrices used in biocomposites:
- Polymer Matrices: These can be either synthetic polymers, such as polypropylene and epoxy resins, or biopolymers, such as polylactic acid (PLA) and polyhydroxyalkanoates (PHA). Biopolymers are particularly attractive for their biodegradability and renewable origins.
- Cementitious Matrices: These include materials like cement, lime, and geopolymer. Biocomposites with cementitious matrices are commonly used in construction applications for their strength and durability.
Biocomposites Manufacturing Methods
Several manufacturing methods are used to combine natural fibers and matrix materials into biocomposites. These methods vary depending on the type of fibers, matrix, and desired properties of the final product. Common manufacturing methods include:
- Compression molding: This process involves placing a mixture of natural fibers and matrix material into a mold and applying heat and pressure to form the composite. Compression molding is widely used for producing automotive parts and construction materials.
- Injection Molding: In this method, the fiber-matrix mixture is melted and injected into a mold under high pressure. Injection molding is suitable for producing complex shapes and high-volume products, such as consumer goods and packaging.
- Pultrusion: Pultrusion is a continuous manufacturing process where fibers are impregnated with resin and pulled through a heated die to form a continuous profile. This method is commonly used for producing structural components like beams and panels.
- Extrusion: Extrusion involves forcing a mixture of fibers and matrix material through a die to create continuous shapes such as rods, pipes, and sheets. This process is used for various applications, including construction and packaging.
- Handled method: The hand lay up method for bio-composite manufacturing is a straightforward and cost-effective technique, ideal for small-scale production and prototyping. This method involves preparing a mold, applying a release agent, and layering natural fibers such as jute, hemp, or flax. Each fiber layer is wetted with a bio-resin, like PLA or soy based resin, using brushes or rollers. Successive layers are added and consolidated to ensure uniform resin distribution and air bubble removal. After all layers are applied, the composite is allowed to cure according to the resin’s specifications, often at room temperature or with heating. Once cured, the composite is demolded and trimmed. The hand lay-up method is advantageous due to its simplicity, low cost, and versatility, making it suitable for a wide range of applications, including automotive parts, aerospace interiors, construction materials, and consumer goods. However, it is labor-intensive, and quality can vary based on operator skill, making it less suitable for high volume production. This method promotes sustainability by using natural fibers and bio-resins, reducing the environmental impact of composite materials.
Example of Biocomposites: Hemp Fiber Composites
Hemp fiber composites are a prime example of biocomposites derived from agriculture. Hemp is a versatile and fast-growing plant that produces strong, lightweight fibers ideal for composite materials. Hemp fibers are used in combination with various matrix materials to create biocomposites with a range of properties and applications.
Properties of Hemp Fiber Composites
Hemp fiber composites exhibit several advantageous properties:
- High Strength to Weight Ratio: Hemp fibers have a high tensile strength, which contributes to the overall strength of the composite while keeping the weight low.
- Thermal Insulation: Hemp fibers provide excellent thermal insulation, making hemp fiber composites suitable for construction and automotive applications where temperature regulation is important.
- Biodegradability: Hemp fibers are biodegradable, reducing the environmental impact of the composite at the end of its life cycle.
- Renewability: Hemp is a renewable resource that grows quickly and requires minimal pesticides and fertilizers, making it an environmentally friendly choice.
Applications of Hemp Fiber Composites
Hemp fiber composites are used in various industries, including:
- Automotive: Hemp fiber composites are used to manufacture interior and exterior components such as door panels, dashboards, and body panels. These composites offer weight savings and improved sustainability compared to traditional materials.
- Construction: Hemp fiber composites are used in building materials such as insulation panels, roofing tiles, and structural components. Their thermal insulation properties and environmental benefits make them an attractive choice for sustainable construction.
- Consumer Goods: Hemp fiber composites are used in the production of consumer goods such as furniture, sporting equipment, and packaging materials. These products benefit from the strength and durability of hemp fibers, as well as their eco-friendly characteristics.
Introducing Biocomposites in Industry
The adoption of biocomposites in industry requires a multifaceted approach involving research and development, market education, and policy support. To successfully introduce biocomposites into various sectors, several key steps must be taken:
Research and Development
Ongoing research and development (R&D) are crucial for advancing the technology and applications of biocomposites. R&D efforts should focus on:
- Material Innovation: Developing new biocomposite formulations with improved properties, such as enhanced mechanical strength, durability, and resistance to environmental factors.
- Processing Techniques: Optimizing manufacturing processes to improve efficiency, reduce costs, and enhance the quality of biocomposites.
- Application Development: Identifying new applications and markets for biocomposites and tailoring materials to meet specific industry needs.
Market Education and Awareness
Educating industry stakeholders about the benefits and potential of biocomposites is essential for driving adoption. This includes:
- Industry Outreach: Engaging with manufacturers, designers, and engineers to demonstrate the performance and advantages of biocomposites through case studies, demonstrations, and technical workshops.
- Certification and Standards: Establishing certification programs and industry standards to ensure the quality and reliability of biocomposites and to build confidence among end-users.
- Consumer Awareness: Promoting the environmental and sustainability benefits of biocomposites to consumers and encouraging demand for products made from these materials.
Policy Support and Incentives
Government policies and incentives can play a significant role in promoting the use of biocomposites. Key policy measures include:
- Subsidies and Grants: Providing financial support for R&D and commercialization of biocomposites, including grants for pilot projects and subsidies for adopting sustainable materials.
- Regulations and Standards: Implementing regulations that encourage the use of sustainable materials, such as biocomposites, in various industries. This can include green building standards, automotive regulations, and packaging requirements.
- Tax Incentives: Offering tax incentives for companies that invest in biocomposite technologies or use biocomposites in their products.
Biocomposites from Agriculture: A New Hope for Employment
The development and adoption of biocomposites can create significant employment opportunities, particularly in rural and agricultural communities. By transforming agricultural by-products into valuable materials, biocomposites can drive economic growth and job creation across multiple sectors.
Job Creation in Agriculture
The production of biocomposites begins with the cultivation and harvesting of natural fibers. This creates demand for agricultural labor, providing new income sources for farmers and rural workers. Additionally, the processing of agricultural residues into fibers involves various stages, such as extraction, cleaning, and treatment, which require skilled and unskilled labor.
Manufacturing and Processing Jobs
The manufacturing of biocomposites involves several steps, including fiber processing, matrix formulation, and composite production. Each of these steps requires a workforce with diverse skills, from technicians and machine operators to engineers and quality control specialists. The establishment of biocomposite manufacturing facilities can create numerous jobs in the regions where these facilities are located.
Research and Development Positions
Ongoing R&D in biocomposites necessitates a skilled workforce of scientists, engineers, and researchers. These professionals are needed to develop new materials, optimize production processes, and explore innovative applications for biocomposites. R&D activities can be carried out in academic institutions, private companies, and government research centers, providing employment opportunities in the science and technology sectors.
Indirect Employment
The growth of the biocomposite industry can also generate indirect employment in related sectors, such as logistics, marketing, and sales. As biocomposites gain market acceptance, there will be increased demand for transportation, distribution, and marketing services to support the supply chain and bring products to market.
Biocomposites from Agriculture: A Move Towards a Green Economy
The transition to a green economy involves adopting sustainable practices and materials that reduce environmental impact and promote resource efficiency. Biocomposites from agriculture are a critical component of this transition, offering numerous environmental, economic, and social benefits.
Environmental Benefits
Biocomposites help reduce the environmental footprint of materials in several ways:
- Reduced Carbon Emissions: By using renewable natural fibers and biodegradable matrices, biocomposites can significantly reduce carbon emissions compared to traditional composites made from fossil fuels.
- Waste Reduction: Utilizing agricultural by-products and residues in biocomposite production helps divert waste from landfills and reduces the environmental burden of waste management.
- Resource Efficiency: Biocomposites promote resource efficiency by making use of renewable and abundant natural resources, reducing the reliance on non-renewable materials.
Economic Benefits
The adoption of biocomposites can drive economic growth and sustainability.
- Value Addition for Farmers: By creating new markets for agricultural by-products, biocomposites provide farmers with additional income streams and enhance the economic viability of farming.
- Job Creation: The biocomposite industry can create numerous jobs across the supply chain, from agriculture and manufacturing to R&D and sales, contributing to economic development and poverty alleviation.
- Innovation and Competitiveness: The development and commercialization of biocomposites can spur innovation and enhance the competitiveness of industries that adopt these materials, leading to economic growth and technological advancement.
Social Benefits
Biocomposites can contribute to social sustainability by:
- Improving Rural Livelihoods: The production and processing of natural fibers provide employment and income opportunities for rural communities, improving livelihoods and reducing rural-urban migration.
- Promoting Health and Safety: Biocomposites often involve fewer harmful chemicals and emissions compared to traditional composites, promoting safer working conditions and reducing health risks for workers and consumers.
- Enhancing Community Resilience: By supporting sustainable agriculture and local economies, biocomposites can enhance the resilience of communities to economic and environmental shocks.
Research and Development in Biocomposites for Industry
Continued research and development (R&D) are essential for advancing the technology and applications of biocomposites. R&D efforts focus on several key areas to improve the performance, sustainability, and market acceptance of biocomposites.
Material Innovation
Developing new biocomposite formulations is a primary focus of R&D. This involves exploring various combinations of natural fibers and matrix materials to achieve desired properties such as strength, durability, and biodegradability. Researchers are also investigating the use of advanced biopolymers and hybrid materials to enhance the performance of biocomposites.
Processing Techniques
Optimizing manufacturing processes is crucial for improving the efficiency and quality of biocomposites. R&D efforts in this area include developing advanced processing techniques, such as 3D printing and nanotechnology, to create high-performance biocomposites with tailored properties. Additionally, researchers are working on reducing energy consumption and minimizing waste in biocomposite production.
Application Development
Identifying and developing new applications for biocomposites is another important area of R&D. This involves collaborating with industry partners to tailor biocomposite materials for specific uses, such as automotive components, building materials, and consumer goods. By demonstrating the performance and benefits of biocomposites in real-world applications, R&D efforts can drive market acceptance and adoption.
Life Cycle Analysis and Sustainability
R&D in biocomposites also includes conducting life cycle analyses (LCAs) to evaluate the environmental impact of biocomposites throughout their life cycle, from raw material extraction to end-of-life disposal. These studies help identify areas for improvement and support the development of more sustainable biocomposite products. Additionally, researchers are exploring the use of recycled materials and waste streams in biocomposite production to further enhance sustainability.
Collaboration and Knowledge Sharing
Collaboration between academic institutions, industry, and government is essential for advancing biocomposite technology. R&D initiatives often involve partnerships and knowledge sharing to leverage expertise and resources. This collaborative approach accelerates innovation and helps address the technical and market challenges facing the biocomposite industry.
Biocomposite Value Addition to the Farmer
The production of biocomposites from agricultural by-products offers significant value addition to farmers, transforming what would otherwise be waste into valuable resources. This value addition can have profound economic and social impacts on farming communities.
Diversified Income Streams
By supplying natural fibers for biocomposite production, farmers can diversify their income streams and reduce their dependence on traditional crop markets. This diversification can provide a more stable and resilient income, helping farmers weather market fluctuations and adverse conditions.
Enhanced Economic Viability
The ability to sell agricultural residues and by-products enhances the economic viability of farming operations. By turning waste into a valuable resource, farmers can improve their profit margins and invest in better farming practices and technologies.
Sustainable Farming Practices
The demand for natural fibers can encourage sustainable farming practices. For example, crops like hemp and flax are often grown with minimal pesticides and fertilizers, promoting soil health and biodiversity. Additionally, the cultivation of fiber crops can provide rotational benefits, improving soil structure and reducing the need for chemical inputs.
Community Development
The development of a biocomposite industry can stimulate economic growth and development in rural areas. By creating jobs and supporting local businesses, the biocomposite industry can contribute to the overall development and prosperity of farming communities.
Environmental Benefits
Utilizing agricultural by-products for biocomposites helps reduce waste and promote sustainable resource use. This can lead to environmental benefits such as reduced landfill waste, lower greenhouse gas emissions, and improved soil health. These environmental benefits can also enhance the long-term sustainability of farming practices.
Access to New Markets
The biocomposite industry can open up new markets for farmers, providing opportunities to sell their products to a wider range of customers. This access to new markets can increase the demand for agricultural by-products and improve the economic prospects of farming communities.
Biocomposites from agriculture represent a transformative opportunity for achieving sustainability in materials science and industry. By harnessing the abundant and renewable resources available in agriculture, biocomposites offer a sustainable alternative to traditional composites with significant environmental, economic, and social benefits. The development and adoption of biocomposites can drive economic growth, create employment, and promote sustainable practices, contributing to the transition towards a green economy. Continued research and development, along with market education and policy support, are essential for realizing the full potential of biocomposites and ensuring their successful integration into various industries. As we move towards a more sustainable future, biocomposites from agriculture stand as a beacon of hope and innovation.