The world’s growing demand for energy, coupled with increasing concerns over climate change and environmental degradation, has sparked an urgent need for innovative, sustainable solutions. Biomass, derived from organic materials such as wood, agricultural waste, and algae, has emerged as a promising renewable energy source. Among various conversion technologies, pyrolysis has gained significant attention for its ability to transform biomass into valuable fuels, chemicals, and materials. This article delves into the principles, applications, and advancements in pyrolysis of biomass, exploring its potential to mitigate climate change, promote energy security, and foster a circular economy.
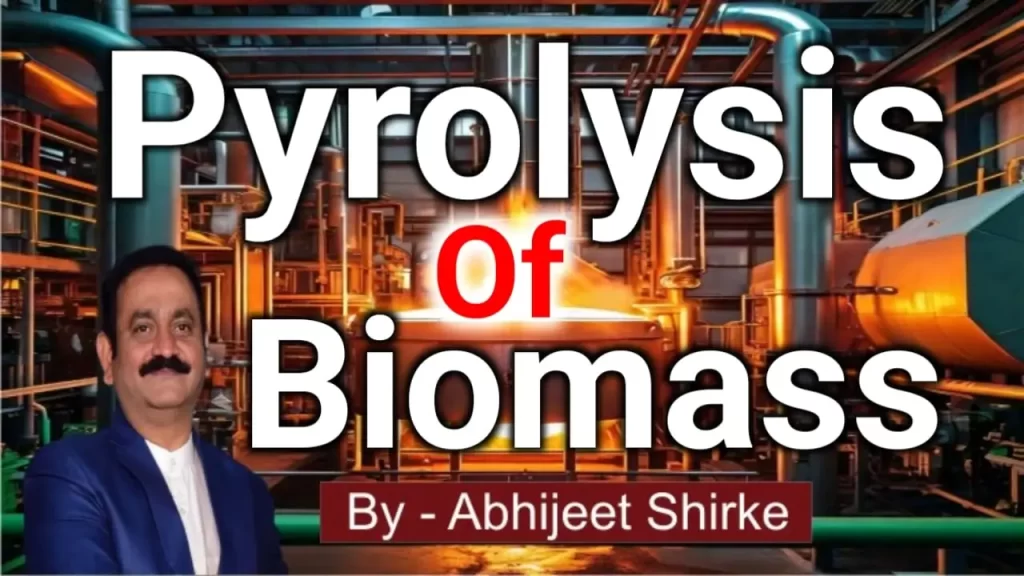
The Biomass Opportunity
Biomass is the oldest and most abundant form of renewable energy, accounting for approximately 10% of global energy consumption. Its potential is vast, with estimates suggesting that biomass can meet up to 20% of global energy demand by 2050. Biomass feedstocks are diverse, ranging from forestry residues and agricultural waste to municipal solid waste and algae. This variety ensures a consistent supply, reducing reliance on fossil fuels and enhancing energy security.
Pyrolysis: A Game Changer in Biomass Conversion
Pyrolysis, a thermal decomposition process, offers a efficient and versatile method for converting biomass into:
1. Bio-oil: a liquid fuel suitable for transportation and power generation
2. Biochar: a solid carbon-rich material for soil amendment and carbon sequestration
3. Syngas: a gaseous mixture for power generation, chemical synthesis, or fuel production
Pyrolysis operates in the absence of oxygen, distinguishing it from combustion and gasification. This anaerobic environment enables the preservation of biomass energy content, minimizing energy losses and maximizing product yields.
Advantages and Challenges
Pyrolysis of biomass presents several advantages:
1. Carbon-neutral energy production
2. Reduced greenhouse gas emissions
3. Waste reduction and valorization
4. Diversified energy portfolio
However, challenges persist:
1. Scalability and cost-effectiveness
2. Feedstock variability and quality
3. Process optimization and control
4. Standardization and regulation
I. Preface
1. Pyrolysis and Its Application in Biomass Conversion
Pyrolysis is a Thermochemical process that involves the decomposition of organic materials in the absence of oxygen, resulting in the production of bio-oil, bio char, and syngas. This process is particularly significant for biomass conversion, transforming a wide variety of organic feed stocks, such as agricultural residues, forestry waste, and municipal solid waste, into valuable energy and chemical products. Biomass pyrolysis offers a versatile method for converting low-value or waste materials into high-value bio fuels and chemicals, contributing to the development of sustainable and renewable energy systems. Its application spans various industries, including energy generation, waste management, and environmental remediation.
2. Importance of Renewable Energy Sources and Biomass Utilization
The growing Global energy demand and the depletion of fossil fuel reserves necessitate the exploration of renewable energy sources. Biomass, a versatile and abundant renewable resource, has gained significant attention due to its potential to produce energy and chemicals sustainably. Utilizing biomass through pyrolysis reduces the reliance on non-renewable resources and contributes to mitigating climate change by lowering greenhouse gas emissions. Additionally, biomass utilization supports waste management practices, providing a circular economy approach by converting organic waste into energy and valuable products.
3. Brief of the Pyrolysis Process and Its Benefits
The pyrolysis process involves heating biomass at elevated temperatures (300°C–800°C) in an oxygen-deprived environment, resulting in its thermal decomposition into three primary products: bio-oil, bio char, and syngas. Each product has distinct applications, such as bio-oil for fuel and chemical production, bio char for soil enhancement and carbon sequestration, and syngas for power generation. The benefits of pyrolysis include energy recovery, reduced carbon emissions, and the production of versatile bio products that can replace conventional fossil-based fuels and chemicals. Furthermore, the process can be adapted to handle various types of biomass, enhancing its appeal as a sustainable technology.
4. Global Energy Scenario and Need for Sustainable Solutions
The global energy sector is at a crossroads, grappling with the dual challenge of increasing energy demands and reducing environmental impacts. Fossil fuel combustion continues to contribute significantly to greenhouse gas emissions and environmental degradation, necessitating a shift toward sustainable solutions. Pyrolysis of biomass presents a feasible option to address these challenges, providing a renewable energy source while also generating valuable by-products. As countries strive to meet climate targets and reduce their carbon footprint, technologies like biomass pyrolysis are essential for transitioning to a low-carbon economy.
5. Pyrolysis of Biomass Offers a Promising Solution for Energy and Chemical Production
Given the increasing emphasis on sustainability and environmental protection, pyrolysis of biomass emerges as a promising solution for energy and chemical production. It provides an effective way to utilize renewable resources, reduce waste, and contribute to carbon sequestration. By converting biomass into bio-oil, bio char, and syngas, pyrolysis not only addresses energy needs but also offers pathways for producing a range of chemicals and fuels. This article explores the principles, feed stocks, products, and applications of biomass pyrolysis, highlighting its potential to contribute to a sustainable future.
II. Principles of Pyrolysis
1. Thermal Decomposition of Biomass into Bio-Oil, Bio char, and Syngas
The pyrolysis process involves heating biomass in an oxygen-free environment, leading to its thermal decomposition into three main products: bio-oil, bio char, and syngas. Bio-oil is a complex mixture of oxygenated organic compounds, primarily used as a liquid fuel or chemical feedstock. Bio char is a carbon-rich solid that serves as a soil amendment, enhancing soil fertility and sequestering carbon. Syngas, a gaseous mixture of hydrogen, carbon monoxide, and methane, can be used for power generation or as a precursor for chemical synthesis. The product distribution depends on the operating conditions, feedstock type, and reactor design, allowing flexibility in tailoring the process for desired outcomes.
2. Temperature and Residence Time Effects on Pyrolysis Products
Temperature and residence time are critical factors influencing the yield and composition of pyrolysis products. Lower temperatures (300°C–500°C) and longer residence times favor bio char production, while higher temperatures (500°C–800°C) and shorter residence times increase bio-oil and syngas yields. Temperature affects the thermal stability of biomass components such as cellulose, hemicellulose, and lignin, altering the product distribution. Residence time, or the duration biomass spends in the reactor, also plays a crucial role in determining the extent of thermal degradation. Optimizing these parameters is essential for maximizing the efficiency of the pyrolysis process and obtaining desired product compositions.
3. Catalysts and Additives Used in Pyrolysis
Catalysts and additives are often introduced during the pyrolysis process to enhance product quality and yield. Catalysts such as zeolites, metal oxides, and activated carbon can promote specific reactions, leading to improved bio-oil properties or increased syngas production. For example, zeolite catalysts can reduce oxygenated compounds in bio-oil, increasing its stability and energy density. Additives like alkali salts and transition metals can influence the breakdown of biomass polymers, further refining the process. The choice of catalyst or additive depends on the target product and feedstock characteristics, making catalyst selection a crucial aspect of pyrolysis optimization.
4. Pyrolysis Reactor Designs and Configurations
Various reactor designs are employed in pyrolysis, including fixed-bed, fluidized-bed, rotary kiln, and auger reactors. Each design has its advantages and limitations based on feedstock type, scale of operation, and product specifications. Fixed-bed reactors are suitable for small-scale applications, while fluidized-bed reactors offer better heat and mass transfer, making them ideal for large-scale production. Rotary kilns and auger reactors provide continuous operation, enhancing process efficiency. Reactor design influences residence time, heat transfer, and product yield, making it a key consideration in developing an effective pyrolysis system.
5. Process Optimization and Control
Optimization and control of the pyrolysis process are essential for achieving desired product yields and quality. This involves fine-tuning parameters such as temperature, heating rate, residence time, and feedstock properties. Advanced control systems using sensors and real-time monitoring can enhance process stability and efficiency. Process modeling and simulation tools are often used to predict product distribution and optimize reactor designs. Additionally, integrating process control with catalyst selection and reactor configuration allows for greater flexibility and scalability, making pyrolysis a viable technology for diverse biomass feed stocks.
III. Biomass Feed stocks for Pyrolysis
1. Woody Biomass (e.g., Forest Residues, Sawdust)
Woody biomass, including forest residues, sawdust, and wood chips, is one of the most commonly used feed stocks in pyrolysis due to its high lignin content and structural stability. These characteristics contribute to higher bio char yields and improved bio-oil quality. Woody biomass is typically sourced from forestry operations, sawmills, and wood-processing industries, making it a readily available and sustainable feedstock. Its uniform structure and lower moisture content compared to other biomass types allow for more controlled pyrolysis conditions, resulting in consistent product outputs. The use of woody biomass in pyrolysis also supports forest management practices by providing an outlet for waste materials, reducing the risk of wildfires, and promoting ecosystem health.
2. Agricultural Biomass (e.g., Corn Stover, Wheat Straw)
Agricultural biomass, such as corn stover, wheat straw, and rice husks, represents a significant source of feedstock for pyrolysis. These residues are by-products of agricultural activities and are often left in the fields or burned, leading to environmental pollution. Utilizing agricultural biomass in pyrolysis not only provides an alternative energy source but also reduces waste and emissions. However, agricultural biomass often contains higher levels of ash and moisture, which can affect the efficiency of the pyrolysis process. Pre-treatment methods like drying and grinding are typically employed to improve feedstock quality and ensure optimal pyrolysis performance.
3. Municipal Solid Waste (MSW) and Sewage Sludge
Municipal solid waste (MSW) and sewage sludge are unconventional but promising feed stocks for pyrolysis. They offer a solution for waste management while producing energy and chemicals. MSW includes organic fractions like food waste, paper, and plastics, while sewage sludge consists of organic matter and nutrients. Pyrolysis of these materials can reduce landfill volumes, recover valuable resources, and minimize environmental impacts. The heterogeneous nature of MSW and the high moisture content of sewage sludge present challenges in feedstock handling and process efficiency. Despite these challenges, advancements in reactor design and pre-treatment technologies have enabled effective utilization of MSW and sewage sludge in pyrolysis.
4. Algal Biomass and Aquatic Plants
Algal biomass and aquatic plants are emerging feed stocks for pyrolysis, offering high growth rates and minimal land use requirements. Algae can be cultivated in various environments, including freshwater, seawater, and wastewater, making it a versatile and sustainable feedstock. The high lipid content in algae makes it ideal for bio-oil production, while the residual biomass can be used to produce bio char and syngas. Algal pyrolysis can also contribute to nutrient recovery and water treatment, providing additional environmental benefits. Research is ongoing to optimize algal biomass conversion through pyrolysis, focusing on enhancing product yield and quality.
5. Comparison of Biomass Properties and Pyrolysis Potential
Different biomass feed stocks exhibit varying properties such as moisture content, ash content, energy density, and chemical composition, which influence their pyrolysis potential. Woody biomass, with its high lignin content, is more suited for bio char production, while agricultural residues are more prone to producing bio-oil and syngas. MSW and sewage sludge
possess a diverse composition, making their pyrolysis products less predictable but potentially more valuable depending on the feedstock’s organic content. Algal biomass, on the other hand, offers a unique profile with high lipid and protein content, favoring bio-oil production. Understanding the properties of each feedstock is crucial for optimizing the pyrolysis process and achieving desirable product yields. Comparing the characteristics of different biomass types enables the selection of suitable feed stocks for specific pyrolysis applications, maximizing resource utilization and process efficiency.
IV. Products from Biomass Pyrolysis
1. Bio-Oil: Properties, Applications, and Upgrading
Bio-oil, one of the primary products of biomass pyrolysis, is a complex mixture of water, oxygenated organic compounds, and small amounts of ash and solids. It is a dark brown, viscous liquid with a lower energy density compared to conventional petroleum-based fuels. Due to its high water and oxygen content, bio-oil is chemically unstable and requires upgrading before it can be used as a fuel. Upgrading processes such as hydrodeoxygenation and catalytic cracking can enhance bio-oil properties by reducing its oxygen content and increasing its energy density.
Bio-oil can be used as a fuel for heating, power generation, or as a feedstock for producing chemicals like phenols, organic acids, and aldehydes. Its versatility in applications makes it a valuable product, especially when fossil fuel prices are high or in regions with abundant biomass resources.
2. Bio char: Carbon Sequestration, Soil Amendment, and Water Treatment
Bio char, a carbon rich material from pyrolysis, is a porous, high surface area material that enhances soil fertility, water retention, and microbial activity. It plays a crucial role in carbon sequestration, storing carbon in soils for years, and is used for water treatment, making it valuable in environmental and agricultural applications.
3. Syngas: Composition, Cleaning, and Utilization
Syngas, or synthesis gas, is a gaseous mixture primarily composed of hydrogen (H₂), carbon monoxide (CO), and methane (CH₄), along with small amounts of CO₂ and other hydrocarbons. The composition of syngas depends on the feedstock and pyrolysis conditions. Syngas can be cleaned and conditioned to remove impurities such as tar, particulates, and sulfur compounds, making it suitable for various applications. It is commonly used as a fuel for power generation in gas turbines or internal combustion engines. Additionally, syngas serves as a feedstock for chemical synthesis, including the production of methanol, hydrogen, and synthetic natural gas (SNG). The flexibility of syngas in energy and chemical applications underscores its significance as a pyrolysis product.
4. Chemicals and Fuels from Pyrolysis Oil Upgrading
Bio-oil derived from pyrolysis can be upgraded to produce a wide range of chemicals and fuels. Through processes such as hydrotreatment, catalytic cracking, and emulsification, bio-oil can be converted into transportation fuels like gasoline, diesel, and jet fuel. It can also be used to produce valuable chemicals such as phenol, benzene, and xylenes, which are precursors for plastics, resins, and adhesives. The development of efficient upgrading techniques is key to expanding the market for bio-oil-derived products and reducing dependence on petrochemical feed stocks. This not only enhances the economic viability of biomass pyrolysis but also contributes to the production of greener alternatives for conventional chemical industries.
5. Economic Viability and Market Analysis
The economic viability of biomass pyrolysis depends on several factors, including feedstock availability, product marketability, and process costs. Bio-oil, bio char, and syngas all have distinct markets, and their value depends on regional demand, regulatory policies, and competition with fossil-based products. The cost of feedstock collection, transportation, and pre-treatment significantly impacts the overall economics of the process. Government incentives, carbon credits, and renewable energy policies can improve the profitability of pyrolysis projects. A comprehensive market analysis is essential to identify opportunities for product commercialization and to develop strategies for scaling up pyrolysis technologies. Establishing partnerships with industries such as agriculture, waste management, and energy can further enhance the economic feasibility of biomass pyrolysis.
V. Applications and Benefits
1. Renewable Energy Generation (Heat, Power, Transportation Fuels)
One of the primary applications of biomass pyrolysis is the generation of renewable energy in the form of heat, power, and transportation fuels. Bio-oil can be combusted directly in boilers or upgraded into liquid fuels for use in engines and turbines. Syngas can be used in combined heat and power (CHP) systems, providing both electricity and thermal energy for industrial processes or district heating. The utilization of pyrolysis products for energy generation not only reduces the consumption of fossil fuels but also decreases greenhouse gas emissions. As the global energy sector transitions towards cleaner sources, biomass pyrolysis can play a vital role in providing sustainable energy solutions, especially in rural and off-grid areas.
2. Chemical Production (e.g., Phenol, Formaldehyde)
Pyrolysis derived chemicals have the potential to replace petroleum-based feed stocks in the production of various chemicals such as phenol, formaldehyde, and acetone. These chemicals are widely used in industries manufacturing resins, adhesives, and plastics. Bio-oil, in particular, is rich in phenolic compounds, making it a valuable source for phenol production. By substituting fossil-based chemicals with bio-based alternatives, biomass pyrolysis contributes to the greening of chemical industries and promotes the development of a bio-based economy. The use of bio-oil and syngas as chemical feed stocks also reduces the environmental footprint of chemical production, aligning with sustainable development goals.
3. Carbon Sequestration and Climate Change Mitigation
Bio char, as a by-product of pyrolysis, is a highly stable form of carbon that can be sequestered in soils for long periods, effectively removing CO₂ from the atmosphere. The application of bio char in soils does carbon sequestration and enhances soil health by improving nutrient retention and water-holding capacity. This dual benefit makes bio char a critical tool in climate change mitigation strategies.
Moreover, the use of bio-oil and syngas as fuels reduces reliance on fossil-based energy sources, further contributing to carbon reduction. Pyrolysis, therefore, provides a holistic approach to managing carbon emissions and promoting sustainable land-use practices. Visit Biomass2Biooil.com for more details.
4. Waste Management and Resource Recovery
The pyrolysis of biomass offers an effective solution for waste management, converting organic waste streams such as agricultural residues, forestry by products, and municipal solid waste into energy and value-added products. This approach not only reduces the volume of waste sent to landfills but also recovers resources that would otherwise be lost. The process minimizes environmental pollution, reduces the need for land filling, and mitigates the emission of harmful gases such as methane. Integrating pyrolysis with existing waste management systems enhances resource recovery, turning waste into a valuable resource and promoting a circular economy.
5. Rural Development and Job Creation
The deployment of biomass pyrolysis facilities can stimulate rural development by creating jobs and generating income in local communities. Rural areas often have abundant biomass resources that can be harnessed for pyrolysis, providing opportunities for feedstock supply chains and facility operation. The development of small- to medium scale pyrolysis plants can support local economies by providing employment in feedstock collection, transportation, processing, and plant management. Additionally, the production of bio char and its use in agriculture can enhance crop yields, contributing to food security and rural prosperity. Promoting biomass pyrolysis in rural regions helps diversify income sources, reduce rural poverty, and support sustainable development.
The pyrolysis of biomass offers a sustainable pathway for energy and chemical production, addressing global energy needs while contributing to environmental sustainability. Its versatility in handling various feed stocks and producing a range of valuable products makes it a promising technology for the future of renewable energy and green chemistry.